Major themes in our work
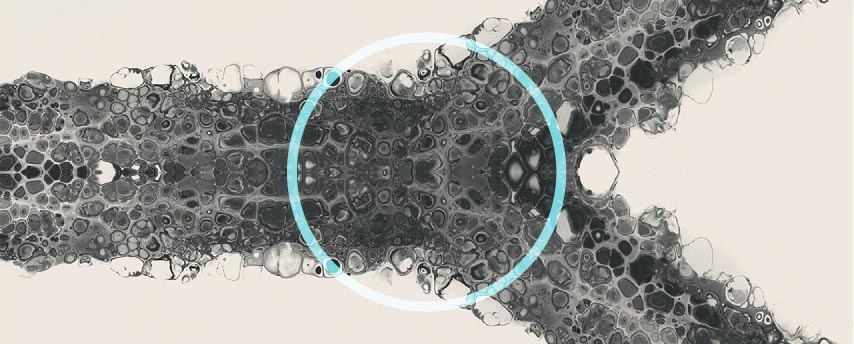
Electron spin-dependent chemical reactions in biology
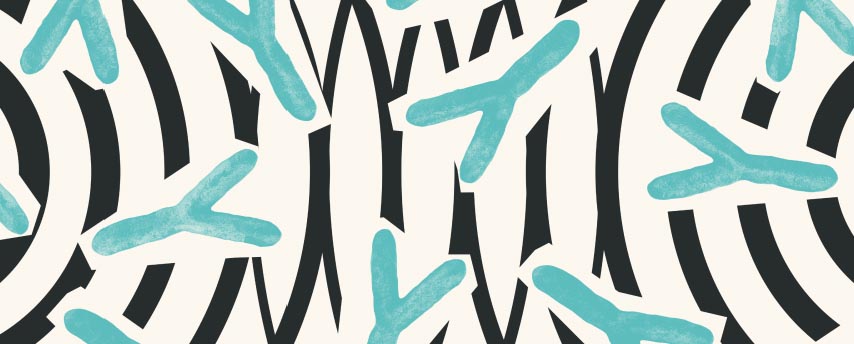
Phonon-assisted molecular recognition
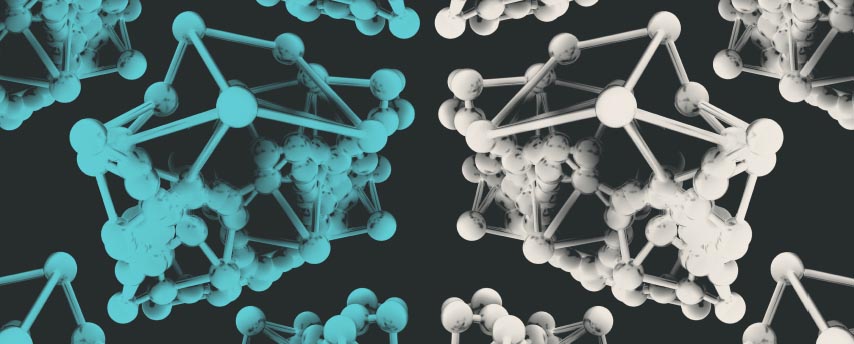
Chiral-induced spin selectivity under a quantum lens
Featured instrumentation
It is possible to detect quantum states optically in proteins. To determine whether these quantum signatures continue to exist in cells requires the development of microscopes that look at weak magnetic field effects in biology with nanosecond time resolution.
Optical spin microscopes
Understanding the role of electron spin-dependent chemical reactions in biology
We are designing and building optical microscopes coupled to coils and radio-frequency microchips, where specimens (from proteins to both invertebrate and vertebrate cells) will be systematically perturbed by magnetic fields. Mid-20th century scientists harnessed the signal provided by biological spins in magnetic resonance imaging—a technology that, to this day, is used for diagnostics. Here, we will go further and influence spins through magnetic resonance to effect treatment. In contrast to magnetic resonance imaging, our technology relies upon very weak magnetic fields (the underlying physical mechanism being different). Our technology will lead to remotely actuated, personalized medicine devices that can predictably alter disease progression using weak magnetic fields.
Electrophysiology spin microscope
Investigating how weak magnetic fields alter ion channel functioning
We will build an electrophysiology microscopy setup coupled to coils and radio-frequency microchips where the following hypothesis can be tested. We hypothesize that spin-dependent chemical reactions happening within the cellular membrane might influence the functioning of ion channels; and that, conversely, transient ion fluxes inside the cell might produce weak magnetic fields to tweak widespread spin-dependent chemical reactions}. Optogenetics relies on laser excitation to control the opening and closing of ion channels in genetically modified cells. Here, with tailored, weak magnetic field excitation and no genetic modification, we posit it might be possible to deterministically affect ion channel functioning— with myriad applications for neurological control.
The ESR-STM
Understanding the role of spin-polarized electron transport in biology
A scanning tunneling microscope coupled to coils and radio-frequency microchips will help decipher why, at room temperature, charge transport through chiral biomolecules favors one spin ("chiral-induced spin selectivity", first observed in DNA and now harnessed in spintronics). Recently, electron transport through a live bacterium nanowire was demonstrated to have such a spin preference, suggesting there is a possibility that other electron transport processes in biology—most importantly, cellular respiration—might also exhibit such a spin preference. From a physics point of view, this is highly unusual: Quantum computers need to spend resources to prepare electrons that favor one spin. Knowing whether and how transport of electronic "quantum information" happens inside chiral biomolecules such as DNA enables the toolkit of quantum physics to be explored towards the control of such quantum information. In practice, this means that quantum methods that control spins via engineered magnetic fields could be applied to tweak physiological processes dependent on electron transport.
The quantum biology revolution is coming
Quantum biology has the power to change the understanding of life around us.
We envision a world where our cell phones can be used to treat medical conditions; where weak magnetic fields can enhance biomanufacturing and inform drug discovery; and where space travel contingencies take into account the presence or absence of weak magnetic fields.
Join us in our mission to elevate the field of quantum biology and develop powerful electromagnetic interventions!

